The “Everything is a Q-Wave” Interpretation of Quantum Physics
Or Say Goodbye to the Wave-Particle Duality…
When we teach quantum physics we still use some old terminology that can actually be very confusing (and may also lead the uninitiated to believe that certain basic things are still not understood!). For instance, the usual brief history of quantum physics goes something like this.
Before quantum there was classical physics. Newton thought that everything is made up of particles. However, various aspects of light could not be explained if light was also made up of particles. Ultimately, Maxwell – standing on the shoulders of Huygens, Young, Faraday and many others – realized that light is actually an electromagnetic wave. At that point the world (still classical) was understood to be made up of two kinds of entities, particles (constituents of matter) and waves (things making up the electromagnetic field). Then came Einstein who (seemingly, but not really) reverted back to Newton and said that light is, after all, made up of particles (photons), though he did admit that they can also behave like waves (which is how we can account for the interference of light). This was the beginning of quantum physics. Finally, de Broglie hypothesized that material quantum objects (such as electrons) can also behave like waves. This led to sort of a unification (everything is a wave and a particle) and inspired Bohr to talk about complementarity, namely the fact that all entities can manifest their particle-like or wave-like nature depending on how we “look at” (experiment with) them. This is at the heart of the famous wave-particle duality of quantum systems.
The situation was so confusing that some prominent scientists were joking that on Mondays, Wednesdays and Fridays electrons were particles, while on Tuesdays, Thursdays and Saturdays they behaved like waves (they were presumably, just like God, resting in an ambiguous state on Sundays). Another standard humorous way of putting it was to say that J. J. Thompson received a Noble Prize for proving that electrons were particles, while his son G. P. Thompson received a Noble Prize (a couple of decades later) for proving that electrons were instead waves (Both were right, so it is not quite clear if this could actually be classified as a joke).
There is fortunately an end to all this talk about waves and particles. Nature is certainly not confused regarding the identity of things, and neither should we be. We no longer need to talk about the wave-particle duality. It is simply a relic of a bygone era.
So, then, what is the right ontology when it comes to reality? Is the world made of particles or waves or things that sometimes behave like particles or waves? According to quantum physics, everything is actually made up of waves (I kid you not). But these are quantum waves (or q-waves for short), meaning that the entities that are doing the waving are what Dirac called q –numbers (as opposed to the ordinary c-numbers, “c” being classical). This picture emerged through the work of Heisenberg, Mott, Schrödinger and Everett (all standing on the shoulders of Hamilton).
Let me explain why everything is a q-wave and why this presents us with the best picture of reality at present. First there was a problem. If particles are waves, the key phenomenon to explain in the 1920s was the observation of the alpha-particle decay in a cloud chamber. This experiment seemed to present a paradox for quantum physics.
An alpha-particle is a Helium nucleus (two protons and two neutrons) and it sometimes gets ejected in a nuclear decay of a larger nucleus. A cloud chamber was a great invention to observe such particles (worthy of several Nobel Prizes), though nowadays you can make one in 15 minutes in your own house with the existing kitchen utensils (there are many YouTube videos on this). The idea, as the name suggests, is to have a particle travel through a gas that can readily be ionized by collisions with the particle. As the particle collides with the gas molecules, it ionizes them in succession. Ionisation attracts neighbouring gas which condenses around the ionized molecules. Therefore, the travelling and colliding particle leaves a track of condensed vapour in its wake (as in the picture below from an actual experiment).
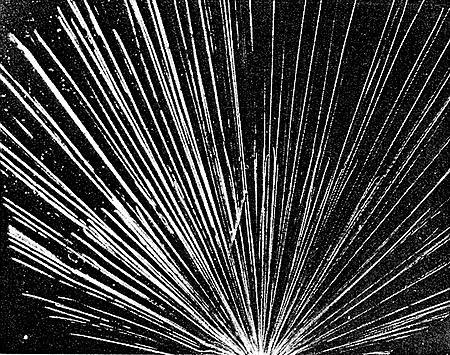
Alpha-particles generated here (at the bottom near centre) and moving though the cloud chamber. Each track belongs to a single emitted alpha-particle.
So far so good, but the problem was that the tracks are always straight lines. If, as quantum physics suggests, everything is a wave, why do we get straight lines from alpha-particles? Why not concentric circles, just like waves spreading in a pond when we throw a stone in it?
Waves in a pond. Why are the images of alpha-particles not like this?Heisenberg was the first person to explain this using his Uncertainty Principle. The emitted alpha-particle, he said, starts out as a wave, but the first molecule it hits localizes it to a small region of space (roughly the size of that molecule). In other words, this collision acts like a measurement of the position of the alpha-particle. But the better we know the position, the less we know the momentum and, so, subsequently, the alpha-particle starts to spread in all directions like a wave. However, the next collision comes pretty soon as the gas in the camber is dense. This again focuses the particle’s position. And so on, the rapid chain of collisions with the gas acts like a sequence of measuring devices that do not allow the alpha particle to spread out like a wave. It therefore leaves a straight track just as a particle would!
It’s a magical explanation, and it seems to make sense. But in 1930 Mott went even further. He actually set the scene for what is now called the Many Worlds Interpretation of quantum mechanics (which should actually be called “Everything is a Q-Wave Interpretation”). Mott said that a single particle simultaneously traverses all the tracks in all directions, meaning that all trajectories exist in a superposition and at the same time. A single alpha-particle takes all the paths simultaneously and in all the directions, it’s just that when we look at it, we can only see one of these trajectories.
The reason for this is that even though everything is a q-wave within which things exist at the same time, when we interact with this q-wave we can only reveal some of its aspects, one at a time (this is where Heisenberg’s Uncertainty comes from). But we ourselves are also a collection of q-waves. It is when our q-waves correlate with the q-waves of the alpha-particle that c-numbers emerge. These correlations between q-waves are called quantum entanglement and so the classical world owns its own existence to quantum entanglement. In quantum physics, even a collision between two particles is actually described as an interaction between two q-waves. This constitutes our most accurate description of nature, called quantum field theory. A particle in this theory is just one stable configuration of the underlying q-wave (or, a single excitation of the quantum field, in a more formal language of quantum field theory).
Schrödinger, in lectures given towards the end of his life, clearly spelt out the same picture that everything is a q-wave. He advocated this view not only because it avoids the confusion arising from the dualistic wave-particle language, but also because it contains no collapses of the wave-function, no abrupt discontinuities due to measurements and no quantum jumps (he was particularly keen to avoid quantum jumps, about which he said that if they turned out to be true he had wished he was a plumber and not a physicist).
Everett usually gets the credit for promoting the view in which everything is quantum and measurements are just entanglements between different quantum systems, however, as I have argued, many other physicist reached the same conclusion (as the famous Schrödinger’s cat thought experiment testifies to). Everett emphasized the relative nature of quantum observations, meaning that relative to my state of being happy, the state of the cat is alive, while – at the same time – there is another simultaneously existing branch (you could also call it a path or a track or what-have-you) of the quantum state in which the cat is dead and I am sad.
Now, let me conclude by explaining why they were all children of Hamilton. Hamilton died well before the birth of quantum physics, so how could he have anticipated all this? This is because Hamilton discovered an ingenious way of doing Newtonian physics that ultimately paved the way to quantum physics.
Hamilton thought of particles moving in straight lines as rays of light moving in a uniform medium. Also, when a force acted on a particle to change its direction of motion, this was for Hamilton analogous to light entering a denser medium and refracting (bending). Hamiltonian mechanics therefore uses the methodology of waves (things like wavefronts, rays, refractive indices etc) to describe the mechanics of particles (things like trajectories, forces, accelerations etc). If we could resurrect him, Hamilton would have no problem understanding the alpha-particle tracks in a cloud chamber. The only thing he was missing at the time were the q-numbers. There simply was no need for them (i.e. no experimental evidence to force us to use them) prior to the twentieth century. Otherwise, Hamilton would have probably written down the Schrödinger equation some fifty years before Schrödinger. This sounds to me like a good idea for an alternative history novel.
Sign up to my substack if you'd like to have my articles delivered straight to your inbox
ASK ME ANYTHING!
If you'd like to ask me a question or discuss my research then please get in touch.